(after James Tiptree’s novel)

[Click to embiggen image]
The flood of data from the solar system probes has led to tectonic shifts in our understanding of our neighbors. High on that list has been the discovery of liquid water in the large moons of the gas giants: Jupiter’s Europa and Ganymede, Saturn’s Enceladus. By many strands of evidence – which include geysers – all three have large subsurface salt-water seas below their icy surfaces (Saturn’s Titan has surface oceans but they’re made of methane and ethane, liquid at Titan’s ambient temperature). In Europa and Enceladus this water may be in contact with a complex silicate core that could supply both directional scaffolding and building blocks; and the orbital friction between the moons and their primaries generates heat dynamos that could give rise to deep hydrothermal vents.
Salt water with a steady supply of dissolved nutrients; an energy source; complex silicates that – according to Cairns-Smith’s clay hypothesis – could act as anchors and template propagators for chiral organic molecules. The simultaneous presence of all these components is a recipe for the development of life.
Life as we know it is based on carbon and uses water as its solvent. Both are unique within their respective categories. Carbon is the best foundational element for complex chemistry by several orders of magnitude: the number and variety of organic compounds exceeds that of all the rest combined. Like its fellow occupants of the fourth column of the periodic table, carbon has an outermost electron orbital that is exactly half-occupied. So the fourth column elements are equally good as electron donors or acceptors, and as a result they can form compounds with just about every other element.
Carbon has an additional almost-unique characteristic: its unoccupied orbital is at such a distance from the nucleus that it can form bonds of the exactly correct strength to create very large and complex compounds. In particular, carbon bonds with itself more or less with the same proclivity that it bonds with anything else. Whatever can be imagined, of any size, shape, taste or smell, can be found among organic compounds, from diamonds to nucleic acids, from limonenes to fullerenes. If a carbon atom’s four available positions have distinct occupants, the resulting compounds are chiral (“handed”), another apparent prerequisite for biomolecules – certainly a decisive attribute of all terrestrial ones.
Silicon, the next foundational candidate after carbon, is a distinctly inferior also-ran. The radius of its outer electron shell is larger, which means that it forms weaker bonds, especially with itself. Generally compounds with more than three silicon atoms in a row are very unstable, unless they are forced into a crystal lattice. And if oxygen is anywhere near it, all available silicon funnels itself into silicates, precluding all other combinations.
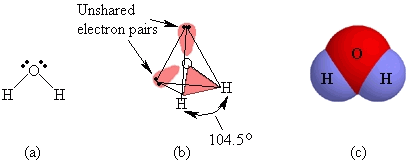
In turn, water has several properties that make it the most potent and versatile solvent. This includes its tetrahedral structure (due to its two free electron pairs, which make it a polar compound), phenomenal heat capacity (which makes it a stabilizer), high heat conductivity and surface tension (vital for many cellular structures and processes), transparency to visible light (crucial for photosynthesis) and the anomalous property of becoming less dense when it solidifies into ice (hence a reservoir and refuge). The runner-up, ammonia, shares some of these attributes (including the tetrahedral structure) and might be the solvent of choice at the lower temperatures of Titan’s hydrocarbon seas. Such a configuration is fully deployed in Joan Vinge’s justly famous Eyes of Amber.
If we encounter life elsewhere, it’s a foregone conclusion that its details will differ significantly from ours – and that its differences will dwarf what SF has come up with. Non-terrestrial life may not use DNA or RNA as its basis of genetic transmission; it may use a different kit of starting blocks for energy, scaffolding and catalysis. It will have a totally different repertoire of body plans, sensoria, mental processes, reproductive modes, ecosystems. But it will be based on carbon and will almost certainly use water as its solvent. And just from current percentages, it’s possible that most planetary life may have developed in “roofed ocean” worlds like Europa, instead of the open atmosphere of Earth.
Walking rightward (as is customary) in the Drake equation, the question is: if they emerge, would roofed-ocean lifeforms evolve to complexity? To sentience? To use of technology, whereby they might send the unambiguously civilizational signals still eagerly awaited by SETI? Of course, our horizons are limited by our own intrinsic parochialisms. We cannot easily visualize technology that’s not based on metals and fire. We cannot easily imagine how sentients that never see the stars might nevertheless deduce their existence.
Many of these perceived hurdles are in fact easily overcome. In Forerunner Foray, André Norton postulated a species that directed the building activity of coral polyps. The solution of water-dwelling lifeforms would be direct-to-biotech, bypassing metal forges. Terrestrial cephalopods are remarkably intelligent and are known to use technology (cetaceans are revenants to water, so their intelligence springs from the same foundation as ours). As for guessing the existence of the stars, a species with sensors in the right bracket of the EM spectrum would rapidly become aware of the overwhelming nearby presence of Jupiter or Saturn. Such species might eventually build starships from tissue, like Farscape’s Moya. Beyond that, the specifics of such species might go a long way towards explaining the over-invoked Fermi Paradox: if they sent signals, they would automatically choose their own waterhole frequency.
So far, we’ve seen the exteriors of roofed-ocean worlds. Missions have been planned for investigations of interiors, though their launch dates keep slipping further into the future. In the end, our own vaunted ability to see the stars may not avail us if we choose to turn inward, eventually running out of the metals and fuels that keep our window to the universe open. If we do send out exploratory vessels, we have to be extra careful not to mar the worlds we touch, that may harbor their own tinkerers and dreamers. But it’s my fond fantasy that, before my own life sets, we get to hear of filigree manta rays swirling under Europa’s ice to the radio pulse beats of the giant overhead.
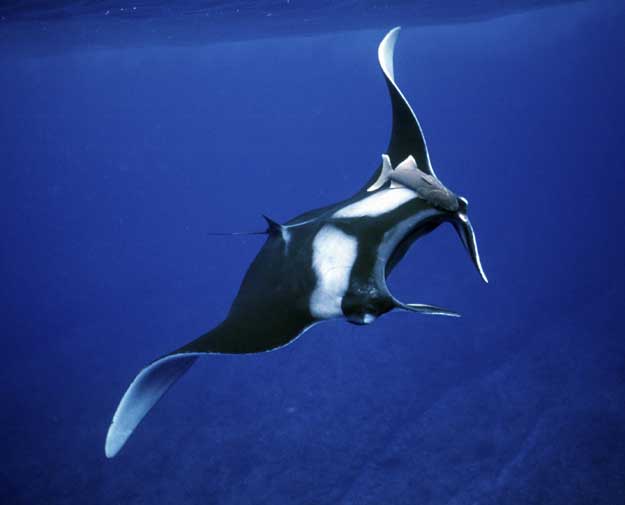
Images: Top, the Europa system (NASA/JPL); middle, water, the marvelous solvent; bottom, a manta ray pirouetting in its element (Getty Images).